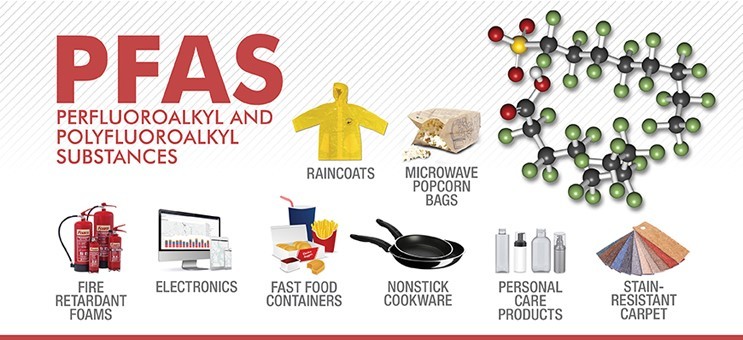
What is New in PFAS: The Nitty, The Gritty, and The Weird
PFAS are an emerging contaminant in the water industry, but there is still much debate on the severity of their presence and how to handle them. These chemicals come in thousands of forms but are all characterized by a highly durable fluoroalkyl chain. Some, such as PFAS, are dubbed “forever chemicals” for their longevity in the natural environment. The obvious culprits of PFAS water pollution are Teflon and firefighting foams, but PFAS can also be found in products such as paint, fast food packaging, water and stain resistant clothing and furniture, some dental floss, and various personal care products such as shampoo. Over 1,200 different forms of PFAS have been used commercially around the world since 1940, and 600 forms are still in commercial production today. Due to the extensive range of PFAS related industries, these chemicals have been found in lakes, rivers, and even in ground water sources. PFAS are also transported in the air and have been found in glacial ice in the Himalayas.
PFAS Chemistry
Poly- and perfluoroalkylated substances (PFAS) are classified as compounds that are fluorinated and contain at least one perfluoroalkyl monomer, a chain of carbons with at least two fluorine molecules attached to each carbon. They are also polar and considered to be amphiphilic which means they are attracted to both lipids and water. Since fluorine is the most electronegative element and carbon greatly favors electronegative charges, the carbon-fluorine bond is among the strongest of organic bonds. A perfluoroalkyl substance usually contains a few perfluoroalkyl monomers while a polyfluoroalkyl substance contains many perfluoroalkyl monomers or is extended to a polymer by other means. The differentiation between the over 4,000 discovered forms of PFAS are characterized by the number of perfluoroalkyl monomers in the chain and by the functional groups attached to the chain. The “forever chemical” PFAS poses a unique issue of treatment and removal because the C-F bonds are not easily degraded. Due to this high level of molecular stability, the PFAS is also classified as “bio accumulative” in the environment. The longer polyfluoroalkyl chains can sometimes be broken down into smaller perfluoroalkyl substances; however, since perfluoroalkyl substances are still a risk, this possible transformation is not desirable (Buck et al. 2011). PFAS are also uniquely difficult to treat because they are surfactants, with a hydrophilic head and a hydrophobic chain. This makes PFAS water soluble on one side of each molecule and oil soluble on the other side of the molecule.
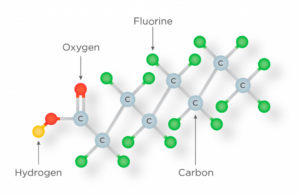
Photo Credit: PFAS Facts
Risks
It is estimated that up to 110 million Americans may be consuming water that is significantly contaminated with PFAS. In November of 2019, the Center of Disease Control and the Agency for Toxic Substances and Disease Registry allocated 7 million dollars to study the medical risks of PFAS (AMA 2019). Unfortunately, in early 2020, a global pandemic changed the course of research for the CDC, and this has greatly slowed the progress of the planned PFAS research. Despite this, the pandemic brought about an unanticipated piece of information regarding the risks of PFAS. Prior to COVID-19, the primary focus of PFAS studies had been in the typical areas affected by commercially produced chemicals: cancer, childhood development, fertility, hormone production, and cholesterol levels. Findings of this research have identified PFAS to increase risk of kidney and testicular cancer, decreased weight of newborns, pre-eclampsia in pregnant women, changes in liver function, and hypercholesterolemia. On the last day of 2020, a study by the Public Library of Science was released that indicated PFAS may have a major role in the severity of COVID-19 in patients with exposure to PFAS. The study identified five different types of PFAS as immunotoxic therefore affecting immune responses to viruses. The study also identified one short chained PFAS, Perfluorobutyrate (PFBA) which accumulates in the respiratory system, as a COVID-19 severity increaser. The study found that, among the hospitalized patients in the study, patients with COVID-19 are 5.18 times more likely to be moved into intensive care or to pass away from the virus if PFBA is present in their plasma. PFAS also has been identified by the CDC to reduce children’s ability to develop the desired antibodies from vaccinations. While further research is required, the EPA has set the PFAS lifetime health advisory at 70 parts per trillion as a preliminary standard for all possible health concerns.
Typical Treatment Methods
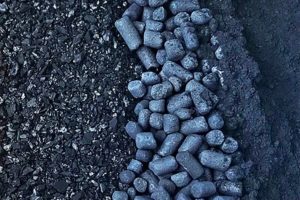
Photo Credit: Evoqua Water Technologies.
Granular Activated Carbon
A common method for PFAS removal is granular activated carbon adsorption due to the existing granular activated carbon infrastructure. Unfortunately, the adsorption sites on many standard granular activated carbon (GAC) medias are typically not effective in removing all PFAS types. Due to the vast variety of shapes and sizes of PFAS molecules, different forms of activated carbon can remove different types of PFAS. Studies show that positively charged granular activated carbon media filters are effective in removing PFAS with net hydrophilic tendencies. The level of removal in these cases can be increased as the GAC micropore surface area increases. For the granular carbon adsorption of PFAS with net hydrophobic interactions, a mesoporous carbon is more effective. The selection of granular activated carbon for the usage of PFAS adsorption should be dependent on an analysis of the types of PFAS present. For example, common PFAS that indicates a net hydrophilic PFAS presence would be PFBA. If a wide range of PFAS types are known to be present, a dual media filter that utilizes both positively charged activated carbon and mesoporous activated carbon would be recommended. PFAS removal by GAC treatment leaves the treatment plant with a backwash saturated with intact PFAS to safely dispose of. Concerns over the GAC’s micropore PFAS removal effectiveness over time leave many questioning the validity of this method for long term treatment solutions.
Membrane Filtration
Microfiltration, nanofiltration, ultrafiltration, and reverse osmosis can function to separate the PFAS from the water. Since PFAS are generally smaller than one nanometer, nanofiltration and reverse osmosis are ideal for PFAS removal. The rejection rate of PFAS using reverse osmosis has been found by studies to be above 99% while the rejection rate of PFAS using nanofiltration has been found by studies to be between 90% and 99%. The removal efficiency of nanofiltration and reverse osmosis was found to increase with pressure and initial flux.
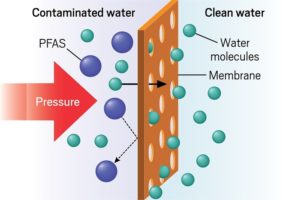
Photo Credit: Designua/Shutterstock/C&EN.
Studies using a commercially produced membrane called polytetrafluoroethylene membrane evaluated the long-term effectiveness of separating PFAS from water. The tests showed that water contaminated with PFAS would “foul” the membrane over a period of a few hours, after which the membrane would reject less and less PFAS, reducing the effectiveness of the membrane long term. The execution of this method resulted in a “plasticization” of the membrane and eventual fracture of the membrane. This fouling is likely due to the membrane’s interaction with the fluorine. Besides choosing a different membrane material that will resist such damage, some possible ways to reduce the chance of fouling would be to provide cyclical descaling and cleaning, to apply an electromagnetic wave, or to purge the membrane with bubbles and rapid backwash. Some other membrane materials that may better withstand PFAS exposure include polyvinyldifluoride membranes and polypropylene membranes because they interact less with the fluorine in the PFAS chains. Like GAC, membranes leave the treatment facility with a concentrated solution of the contaminants for which safe disposal must be considered.
Unique treatment methods
Since PFAS has picked up massive attention recently, many research facilities and industrial treatment vendors are scrambling to develop the next best thing in PFAS removal. Only time will tell the feasibility, long term effectiveness, and operational realities of these new developments. Regardless, it is always exciting to be witness to innovation. In pursuance of that, the following will detail some of the most interesting, and weird, treatment methods in research and development.
Zeolites
Zeolites are a naturally occurring mineral that can be found in areas with alkaline ground water and volcanic activity. They have a crystalline porous structure which make them ideal candidates for adsorption removal of water contaminants. They are naturally hydrophobic and can be used to remove polar molecules but can also be treated to attract non-polar molecules. They are also “molecular sieves” which means they have a uniform structure in their pores that can effectively sort molecules by size. Studies have found certain zeolites to be highly effective in the removal of PFAS from water, even with the presence of other contaminants, due to the thermodynamic attraction of the polar molecules to adsorption sites and the unique pore pattern. While zeolites are more expensive than most adsorption media, they are expected to have extremely reduced lifetime operations and maintenance cost. Since zeolites are minerals, they can withstand heat of 350oC, which is the temperature required to eliminate all PFAS and other contaminants from the crystalline structure. This attribute means the zeolite media could have a very long useful lifetime in removing PFAS. The implementation of this system would require the design of a furnace to clean the media as opposed to a traditional backwash. A backwashing method may also be possible with zeolite filtration.
Biomaterials
A biomaterial adsorbent called chitosan beads is derived from crustacean exoskeletons processed using acetic acids. These beads are highly porous and can provide a high level of negatively charged PFAS adsorption for highly acidic water. The acidic water (3 pH) is required for this method of removal to protonate the amino acids in the chitosan beads and provide an adsorption site for the negatively charged PFAS. These materials can be recycled from marine agriculture byproducts, so they are very cost effective relative to other adsorbents studied. The final adsorption capacity of the chitosan beads was found to be significantly higher than other commonly used adsorbents.
Other biomaterials that can be used for PFAS adsorption include straw and willow char, corn straw ash, and carbon nanotubes. Corn straw ash and carbon nanotubes have been observed in studies to provide attraction to the hydrophobic tails of PFAS and allow for high levels of removal for PFAS. Carbon nanotubes were somewhat more effective in providing removal in this manner, but corn straw ash is significantly more accessible as it is easily derived by incinerating agricultural waste.
Organically Modified Silica
Adsorption via filtration using an organically modified silica can be an effective process for removing certain forms of PFAS. A company called ABS Materials developed two products that can provide adsorption removal using organically modified porous silica materials. These materials swell as they accumulate materials which increases their adsorption capacity. One of the products, Osorb®, is a bulk adsorbent. Another product available, PuraSorb®, is a film placed over a sand and porous silica media. Unfortunately, the adsorption of PFAS by these media varies by the length of the chain, the functional groups on the PFAS, the molecule size, and other factors such as pH and co-contaminants. This method can be very effective in removing longer polyfluoroalkylated substances, but less effective for shorter perfluoroalkylated substances such as PFAA. The removal of shorter chain PFAS such as PFAAs using organically modified silica can be enhanced by decreasing the influent pH.
Biodegradable Surfactant
Surfactants are similar to PFAS in that they are composed of hydrophilic heads and hydrophobic chains. Many surfactants form micelles, a spherical arrangement of the surfactant molecules. Since surfactants mimic the behavior of PFAS, micelles are able to physically adsorb PFAS at a microscopic level, orienting the hydrophobic end of the chain toward the center of the micelle in a similar fashion to the surfactant molecules. Studies have identified a safe, biodegradable surfactant called 1-decanoyl cysteines of April 5th, 2021.The surfactant would absorb the PFAS and then generate a foam. Further evaluation of how this method would be implemented on a treatment scale is still in development. Tests using the surfactant on PFAS contaminated water have seen a 72% reduction of PFAS as well as up to 99% removal of toxic metal ions.
Ozofractionation
Ozofractionation uses the surfactant nature of PFAS molecules to aid removal of PFAS by usage of ozone bubbles. Since PFAS have a hydrophobic perfluoroalkyl chain, this hydrophobic side of the PFAS molecule will attach to the surface of an ozone bubble released in the water. This method requires the release of ozone bubbles from the bottom of a tank. As the ozone bubbles move toward the surface of the water, the bubbles will accumulate the PFAS. Then, the bubbles will come out of the surface of the water as a PFAS dense foam that can be removed from the surface of the water. This foam is hazardous and will require a special disposal plan. A commercially patented process has been developed by Evocra called Ozofractionative Catalyzed Reagent Addition (OCRA) which puts the water through a series of tanks that are saturated with microscopic ozone bubbles to maximize the surface area of the bubbles for the PFAS to bind to. OCRA has been identified to remove 98.6% of total PFAS in the water sources tested without the usage of an additional adsorbent or filter step.
Treatment by Destruction
While the C-F bonds in PFAS are generally too strong for standard biodegradation methods, there are some methods in development that destroy the PFAS. Treatment of PFAS contaminated water by destruction is still in experimental and development stages. Destruction removal processes tend to be energy intensive and expensive. With such expenses, it is recommended that destruction methods be used on the secondary waste streams coming out of adsorption removal media during backwash or out of the concentrate from separation methods.
Sonolysis
Starting in 2009, sonolysis has been utilized to destroy PFAS in raw water in laboratory settings. To treat PFAS contaminated water using sonolysis, sound waves are emitted through the water to induce cavitation. Due to the hydrophobic head of the PFAS molecules, the PFASs will bind to the surface of the bubble created by cavitation. During sonolysis induced cavitation, the temperature of the bubble surface will be around 5000oF which is well above the heat level required to destroy the PFAS molecule. Sonolysis is expected to be effective for destruction of long chain PFAS and short chain PFAS such as PFAA.
Electrochemical Treatment
Studies using electrochemical treatment of PFAS started in 2015, so this technology and the effectiveness thereof is still in development. Electrochemical treatment destroys PFAS by transferring electrons through an electrolyte media between an anode and a cathode. This method is extremely energy intensive, and a residence time of up to 10 hours to removal of all types of PFAS. This treatment method can create toxic byproducts such as chlorine gas, hydrogen fluoride, and perchlorate.
Fungal Degradation
The breakdown of PFAS has been observed in fungal studies using Phanaerochete chrysosporium. The treatment resulted in a 45% decrease of existing PFAS concentrations over a 35-day period, but also saw an increase in some shorter chain PFAS such as PFBA. Other studies used laccase, lignin peroxidase, and manganese peroxidase to aid PFAS degradation using enzyme catalyzed oxidative humification reactions. Studies using this method saw a 30% reduction in PFOA and PFOS which are among the most common PFASs. While this strange method may never reach a water treatment facility, it could potentially be used for PFAS waste disposal facilities.
Degradation in a Plasma Reactor
Another PFAS destruction method was studied by Clarkson University for mobile treatment that uses argon gas, a high voltage discharge from an electrode, and a rotating spark gap motor to create plasma in the water. The plasma interface destroys the long chain PFAS such as PFOS and PFOA but produces short chained PFAS such as PFBA. While the method reduces PFOA and PFOS by around 90%, it also increases PFBA by 562%. This method is extremely energy intensive and will be expensive to operate due to the use of argon gas and high voltage electricity.
Final Remarks
We are constantly learning about PFAS and the multifaceted issues they present. While there is much we don’t know, it’s certain that action will be required in some capacity. Those of us in water resources may be all too excited to call on the treatment methods we already know and love such as GAC and membrane treatment, but the unpredictability of PFAS behavior may require adjustments along the way. We just may need to keep an eye on the research community for some unlikely underdogs, such as crustacean byproducts or exploding bubbles, to save the day.
References
Chen, X., Vanangamudi, A., Wang, J., Jegatheesan, J., Mishra, V., Sharma, R., Gray, S., Kujawa, J., Kujawski, W., Wicaksana, F., & Dumée, L. (2020). Direct contact membrane distillation for effective concentration of perfluoroalkyl substances – Impact of surface fouling and material stability. Water Research (Oxford), 182, 116010–116010. https://doi.org/10.1016/j.watres.2020.116010
Cheremisinoff, N. (2016). Perflourinated Chemicals (PFCs) : Emerging Contaminants of Concern. John Wiley & Sons, Incorporated.
Holmes, T., & Norris, N. (2019). PFAS Legislation. Journal – American Water Works Association, 111(7), 11–11. https://doi.org/10.1002/awwa.1319
Horst, J., McDonough, J., Ross, I., Dickson, M., Miles, J., Hurst, J., & Storch, P. (2018). Water Treatment Technologies for PFAS: The Next Generation. Ground Water Monitoring & Remediation, 38(2), 13–23. https://doi.org/10.1111/gwmr.12281
Kucharzyk, K., Darlington, R., Benotti, M., Deeb, R., & Hawley, E. (2017). Novel treatment technologies for PFAS compounds: A critical review. Journal of Environmental Management, 204(Pt 2), 757–764. https://doi.org/10.1016/j.jenvman.2017.08.016
Kuehn, B. (2019). PFAS Water Contamination Examined. JAMA : the Journal of the American Medical Association, 322(18), 1757–1757. https://doi.org/10.1001/jama.2019.17263
LaFrance, D.B. (2019), PFAS 101. J Am Water Works Assoc, 111: 10-10. https://doi-org.proxy.lib.utk.edu/10.1002/awwa.1318
Rahman, M., Peldszus, S., & Anderson, W. (2014). Behaviour and fate of perfluoroalkyl and polyfluoroalkyl substances (PFASs) in drinking water treatment: A review. Water Research (Oxford), 50, 318–340. https://doi.org/10.1016/j.watres.2013.10.045
Singh, R., Fernando, S., Baygi, S., Multari, N., Thagard, S., & Holsen, T. (2019). Breakdown Products from Perfluorinated Alkyl Substances (PFAS) Degradation in a Plasma-Based Water Treatment Process. Environmental Science & Technology, 53(5), 2731–2738. https://doi.org/10.1021/acs.est.8b07031
Vandermeyden, C., & Hagerty, V. (2020). Managing PFAS: A North Carolina Utility Story. Journal – American Water Works Association, 112(10), 10–18. https://doi.org/10.1002/awwa.1590
Wanninayake, D. (2021). Comparison of currently available PFAS remediation technologies in water: A review. Journal of Environmental Management, 283, 111977–111977. https://doi.org/10.1016/j.jenvman.2021.111977
Ziaee, F., Ziaee, M., & Taseidifar, M. (2021). Synthesis and application of a green surfactant for the treatment of water containing PFAS/ hazardous metal ions. Journal of Hazardous Materials, 407, 124800–124800. https://doi.org/10.1016/j.jhazmat.2020.124800